How Emmanouil Metzakopian is finding gene targets to treat neurodegeneration
Emmanouil Metzakopian is a group leader at the UK Dementia Research Institute at the University of Cambridge, as well as VP of Research and Development at bit.bio. He leads a number of neuroscience projects at Open Targets, one of which recently published a genetic screen aimed at finding genes whose inhibition could protect neurons against cell stress [1].
I asked him to tell me more about the project and its implications for target identification and prioritisation in neurodegenerative diseases.
The main findings
In this project, the team created a Cas9 iNeuron line to perform a genetic screen, and used a CHOP gene reporter to measure the levels of tunicamycin-induced endoplasmic reticulum (ER) stress the cells were experiencing. From this, they validated KAT2B as a gene that, either through CRISPR-Cas9 knockout or pharmacological inhibition by L-Moses, is neuroprotective against ER stress.
How did you get the idea for this project?
The main characteristic of neurodegenerative conditions like Alzheimer’s disease and Parkinson’s disease is the accumulation of misfolded proteins, which leads to cell stress, and particularly relevant for this project, endoplasmic reticulum (ER) stress.
This activates a mechanism called the Unfolded Protein Response through which, if the damage is too great, the cell dies and the neurons are lost. One treatment angle for neurodegenerative diseases is to dampen the Unfolded Protein Response to rescue the neurons.
As a fellow at the Wellcome Sanger Institute, I was trained in gene and cell engineering and genetic screening approaches, including CRISPR-Cas9. Soon after starting my lab, I undertook a project with Open Targets to identify genes that modulate the expression of CHOP, a key gene in the Unfolded Protein Response.
But in fact, the project that was just published — carried out by Sofia Pavlou, a postdoctoral fellow in my lab — was an interesting offshoot of our initial idea, that we decided to pursue as a side project.
What were you hoping to find?
The aim of this project was to find genes that protect neurons from toxic ER stress. This complements previous work I have done on other stress pathways such as oxidative stress and lipid peroxidation. Tunicamycin is a compound that causes the accumulation of unfolded proteins within the cell, leading to ER stress.
If we can find a gene that, when knocked down or knocked out, leads to survival or adaptation to higher stress levels in the cell, then this gene becomes a potential target for a drug that protects cells, especially neurons, against cell stressors.
To our knowledge, this is the first screen performed in iPSC-derived neurons aiming to identify knockouts that are neuroprotective against tunicamycin.
What was your biggest challenge?
Our biggest challenge was to select a suitable model to screen for protective genes. We wanted this to be as close to the situation in humans as possible, so we used iPSC-derived glutamatergic neurons — so-called iNeurons — reprogrammed using the OPTi-Ox technology from Mark Kotter’s lab [2]. We have previously shown that, compared to direct differentiation, reprogramming creates a homogeneous population of cells with fewer batch to batch differences [3]. This homogeneity makes it easier to identify disease-relevant signals in the data.
In fact, the speed and ease of this process surprised us. We didn’t expect reprogramming to be so much more straightforward than direct differentiation, and so much faster. We had our cells in days, in a couple of weeks, compared to months.
The other advantage of reprogramming is scalability. You need hundreds of millions of cells to screen the whole genome; to screen even just the druggable genome requires tens of millions of cells.
The Druggable Genome
The Druggable Genome is the subset of all human genes encoding proteins that could be targeted with a small molecule or antibody drug[4]. This includes genes that are already targets of approved and clinical-phase drugs, genes similar to approved drug targets, genes encoding secreted and extracellular proteins, and members of key druggable gene families.
How did you carry out the project, and what did you find?
Once we had our cell model, we adapted it for screening by knocking Cas9 into a housekeeping gene: a gene that is highly expressed in cells, which is unlikely to be silenced, and which was maintained during the reprogramming process. We then designed our own CRISPR guide RNA library against the druggable genome and transduced the corresponding lentiviruses into millions of our iNeurons. After treating the cells with tunicamycin to induce ER stress, we looked for guide RNAs that were enriched in the cells that survived treatment compared to untreated cells.
From this, we identified 188 genes trending in the right direction, and focused on the 13 that were statistically significant, looking for small molecule inhibitors that could mimic the effect of the knockout. We found L-Moses, a chemical probe targeting KAT2B.
Treating cells with a combination of tunicamycin and L-Moses reproduced the results of the CRISPR-Cas9 screen: pharmacological inhibition of KAT2B protected the cells against tunicamycin-induced ER stress in a dose-dependent manner.
KAT2B has two functions within the neurons: it creates epigenetic modifications in the genome (acetylation), and it acts as a transcription factor. Using transcriptomics and proteomics, we showed that L-Moses affects KAT2B’s transcription factor activity. In fact, there was an overall lower level of transcription in the cells treated with L-Moses.
Looking further, by studying the expression levels of CHOP using a fluorescent reporter, we found that CHOP levels were lower in tunicamycin-treated cells if they were also treated with L-Moses. In other words, the cells treated with L-Moses survived because they were experiencing lower levels of ER stress.
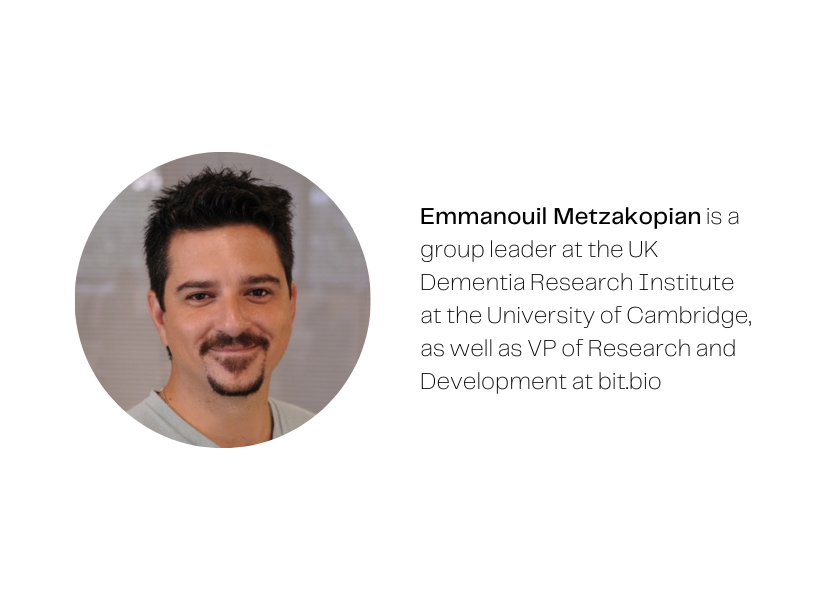
What are the next steps in this research?
One experiment we didn’t get a chance to do was to test L-Moses on mouse models of disease, and human organoids, to see whether it would be a suitable drug to delay or halt neurodegeneration, especially for conditions where we know ER stress is involved. I hope that someone reads this work and tries it, and you never know — it would be amazing if our work led to something that is protective against neurodegeneration.
More broadly in this field, while signs of neurodegeneration appear later in life, we don’t know when it actually starts. The field is actively working on identifying good biomarkers and genetic tests for susceptibility to disease, so that we can find a window in which treatments can reduce the chance of developing dementia, rather than treating the symptoms once they present.
Once we have defined biomarkers and known susceptibility genes, we can begin to stratify patients and have more targeted clinical trials. And so if L-Moses is ever tested in humans, it can be given to specific groups of patients where it has the best chance of alleviating neurodegeneration.
Is there anything else you would like to add?
I would like to say the reason we were able to publish such work is thanks to Open Targets, the fantastic interactions and enthusiasm of the partners, and the support you provide your researchers from start to end. For that, Sofia and I just want to say a big, big thank you.
References
1. Pavlou S, Foskolou S, Patikas N, et al. CRISPR-Cas9 genetic screen leads to the discovery of L-Moses, a KAT2B inhibitor that attenuates Tunicamycin-mediated neuronal cell death. Sci Rep13, 3934 (2023) doi: 10.1038/s41598-023-31141-6 (Open Access)
2. Pawlowski M, Ortmann D, Bertero A, et al. Inducible and Deterministic Forward Programming of Human Pluripotent Stem Cells into Neurons, Skeletal Myocytes, and Oligodendrocytes. Stem Cell Reports. 2017 Apr 11;8(4):803-812. doi: 10.1016/j.stemcr.2017.02.016. (Open Access)
3. Fernandes HJR, Patikas N, Foskolou S, et al. Single-Cell Transcriptomics of Parkinson’s Disease Human In Vitro Models Reveals Dopamine Neuron-Specific Stress Responses. Cell Reports. 2020 33(2). doi: 10.1016/j.celrep.2020.108263.(Open Access)
4. Hopkins, A., Groom, C. The druggable genome. Nat Rev Drug Discov 1, 727–730 (2002). doi:10.1038/nrd892